By Peter Stones
Q: Do you have any tips for selecting the correct grade of stainless filler metal? There seem to be so many different options for every grade, and why is ferrite level so important?
A: The process of choosing a stainless filler metal involves matching it to the base materials, selecting a shielding gas and often calculating ferrite content and total heat input. With many possible combinations of materials, the best choice might not be apparent, especially for those not familiar with the metallurgical nuances. For example, joining 304L to 316L (a common application) presents several filler metal options:
■ 316L (19% Cr, 12% Ni, 3% Mo, 0.4% Si). A perfectly good choice, but the weld pool will be comparatively sluggish, making it more difficult to weld.
■ 316LSi, which has the same composition as above, but with 0.9% silicon. The small, additional silicon content improves wetting and makes the weld pool more fluid and easier to manipulate.
■ 309LSi (24% Cr and 13% Ni) can enhance corrosion resistance by “over-matching” chromium content compared to the other options.
■ Generally, the higher alloyed of the two base metals should be used. In this example, 308L (19% Cr, 9% Ni) or 308LSi (19% Cr, 9% Ni, 0.9% Si) could be used, but it is not an optimum choice.
Selecting the best filler metal also requires understanding service conditions such as corrosive environment, temperature and required mechanical properties. For example, in nitric acid, a 310L filler (which has no molybdenum) will perform better than a 316L or 310LMo due to selective attack of the molybdenum by this specific acid.
Cost also influences filler metal selection. Fabricators use 309L to join 400 Series ferritic alloys, but the high alloy content of 309L (24% Cr and 13% Ni) adds cost. A modified 307 filler, with 8% Ni and 7% Mn, might be a lower-cost alternative because manganese costs less and acts as an austenitizing agent to create a suitable crack resistant microstructure in the weld metal.
Shielding gas
Whether to simplify cylinder management or reduce cost, fabricators often try to use the same shielding gas as they do for MIG or Flux-Cored welding of carbon steel. It does not work with stainless grades because shielding gases with a carbon dioxide (CO2) content of 5% or more add too much carbon to the weld metal, degrading its corrosion resistance. Recommendations for short circuit MIG welding stainless include “tri-mix” gases that contain a blend of 85 to 90% He, up to 10% Ar and 2 – 5% CO2; alternatively Ar + 1-2% CO2 gives good results. A common choice for spray transfer MIG is Ar + 1-2% O2. Options include Ar + (1-2% CO2); Ar + 30% He + 1-2% O2; and Ar + 30%He + 1-2% CO2. Adding O2 gives the weld pool better fluidity and provides arc stabilization; helium gives better weld penetration and increases fluidity of the weld pool and CO2 increases and broadens penetration.

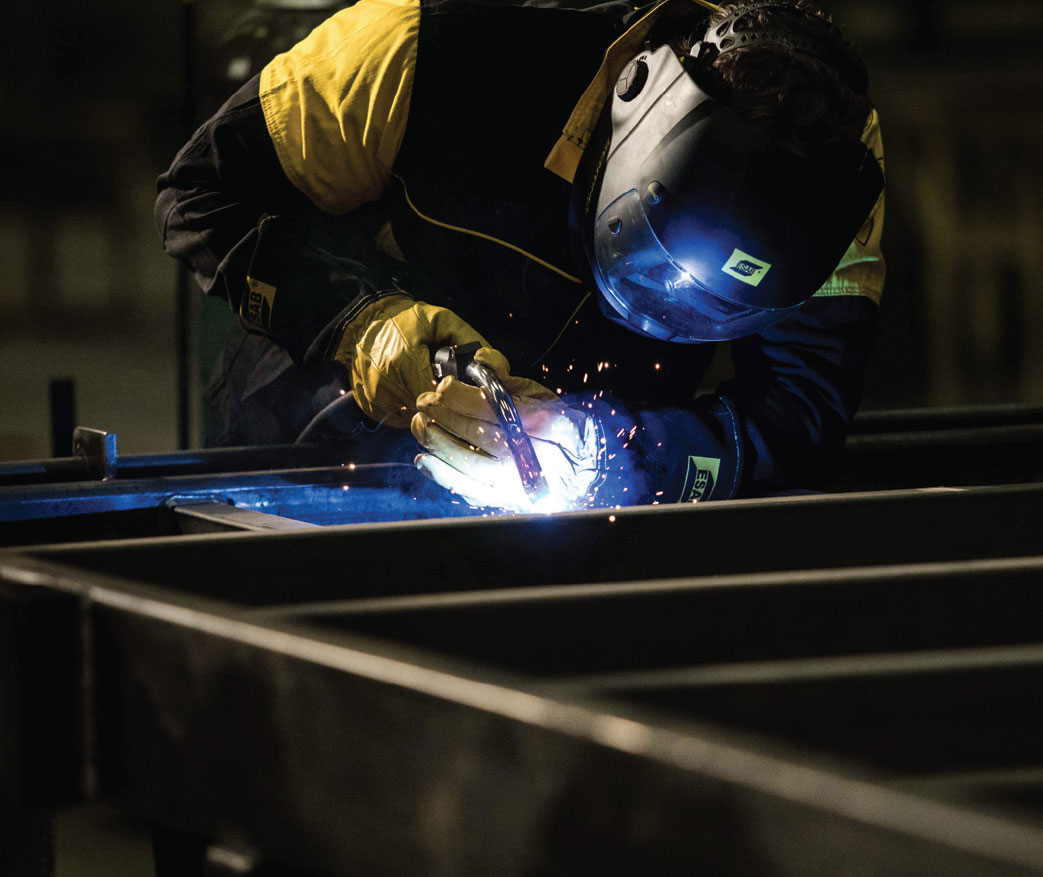
Ferrite calculation
Welding standard austenitic stainless steels requires keeping the ferrite level high enough so that it resists cracking but low enough to prevent transforming to a brittle phase or possible selective attack. To determine balance between the austenite and ferrite forming elements in iron, engineers use one of three constitution diagrams: Schaeffler (the original), DeLong or WRC-1992, and it is the latter one that is the most preferred.
Static charts are widely available, but online and mobile applications allow users to easily switch between diagram options, enter variables (e.g., percentage of carbon, chromium, molybdenum, nickel, nitrogen, etc.) and obtain results.
Heat input
To preserve metallurgical properties, some applications require calculating heat input, which may be a maximum, minimum, or both. For example, with a 2205 duplex alloy, welding below the minimum heat input can result in the wrong phase balance after welding, while excess heat can also affect the corrosion resistance or embrittle the metal. Measured in kJ/mm, heat input requires knowing the values of current, voltage, weld length and arc time. The formula is:
Heat Input = (Amps x Volts) / (1,000 x travel speed in mm/s) = kJ/mm
Over-welding often causes distortion and excess heat input. Rather than reducing volts or amps, a better solution could be increasing travel speed to yield a more ideal bead profile. Some stainless filler metal grades have existed for years, but new ones are being developed to meet the requirements of changing metallurgies, more extreme service conditions, higher yield strength and cost constraints. As a result, even experienced fabricators can benefit by consulting a filler metal expert and leveraging resources such as filler metal data books and online applications.
Meet the columnist
Peter Stones – IEng MWeldI IWE/EWE
As part of the ESAB Specialty Alloys Group, Peter is technical support for stainless and nickel alloy filler metals. Peter is actively involved with TWI and is a non-executive director of The Welding Institute. Peter worked for Sandvik for 10 years and was Global Product Manager for Sandvik Welding up to 2018, when ESAB purchased the filler metals business. Peter.stones@esab.com