Biogas plant in Australia, modernized by Weltec. With food waste as feedstock, the electricity generated powers both the facility itself and a sewage treatment plant, with excess energy exported to the electricity grid.
Bioenergy, the generation of power from organic matter, is a major and growing industry that plays a crucial role in the circular economy. However, its undoubted advantages come with a few drawbacks, including its high costs. An important trend is that the industry is turning increasingly to duplex stainless steel to reduce costs and ensure strength and corrosion resistance.
By James Chater
Background
It may surprise advocates of solar and wind energy, but bioenergy accounts for 55% of renewable energy and over 6% of the world’s energy supply, or five times the combination of wind and solar PV (1). This sector of the renewable energy industry has been growing rapidly and has received a further boost from rising energy prices following the Russian invasion of Ukraine, sanctions on its oil and gas exports and the Europeans’ replacement of Russian piped gas with more expensive LNG from the USA. The IEA considers bioenergy to be an important part of the transition to net zero emissions. Demand is rising more quickly in Brazil, India and Indonesia than elsewhere. All have abundant feedstocks and are net energy importers. Not only these countries but also North American and European governments have programs and directives in place to boost bioenergy production with the help of subsidies and incentives: for example, the USA’s Higher Blends Infrastructure Incentive Program.
Biomass
It is usual to distinguish three generations of biomass (the organic feedstock used to create biofuels):
- Edible crops such as corn, sugarcane, palm oil or soybeans, which are used to make ethanol.
- Non-food biomass such as waste (agricultural or domestic), wood, switchgrass, etc. For example, several pilot projects are scaling up production of cellulosic ethanol, especially in the USA and Europe.
- Algae, which can be cultivated on non-arable land and have higher oil content, making them idealfor biodiesel and jet fuel. Algae production has recently gained renewed interest through efficiency gains, for instance closed-loop systems that recycle water and nutrients. It is also gaining interest as a means of wastewater treatment through biosorption, bioaccumulation and intracellular degradation.
Biofuels
Some of the most common biofuels include:
- Ethanol. The most common biofuel, produced through the fermentation of sugar and starches and cellulose (second generation). It can be blended with gasoline. An important producer is Brazil, where ethanol is made from cellulosic biomass such as bagasse.
- SAF. Sustainable aviation fuels are a viable solution for aircraft, where electrification is a very limited option. It can be produced from waste oils, agricultural residues and CO2 captured from the atmosphere. United, Delta and Lufthansa are among the airlines committed to using SAF, and Shell and BP are building facilities to produce it.
- Biodiesel is produced from oils or fats using the chemical reaction transesterification. Along with LNG and hydrogen, it is a promising candidate fuel for HGVs and large ships. It can be blended with traditional diesel. In 2002, West Central (later Renewable Energy Group) opened one of the world’s biggest continuous-flow processing plants for processing soybean oil into biodiesel fuel.
- Biogas (biomethane) is a mixture of methane and CO2. Its feedstock is normally waste or agricultural residues, and one of its most common uses is in waste to energy (WtE). Bacteria break down the feedstock in the absence of oxygen (anaerobic digestion) to produce biogas, which can be injected into the gas grid or used to generate heat and/or electricity (combined heat and power). A byproduct of the process, called digestate, is a good natural fertilizer.
- Syngas, or synthetic gas, consists of hydrogen and carbon monoxide, often with some CO2 and methane. It can replace gasoline and is also used to produce ammonia or methanol.
Table. Major biofuel and district heating projects
Date announced | Description |
31/8/2023 | POET reopens its refurbished bioprocessing facility in Cloverdale, Indiana, USA. |
3/2024 | Hitachi Energy wins contract to recover and repurpose excess heat from data centers to heat various premises belonging to Fortum, including homes, public facilities, and businesses. |
22/5/2024 | Copenhagen Malmö Port (CMP) and Green2x partner to build one of the world’s largest biofuel plants in the port of Malmö, Sweden. |
13/8/2024 | Verbio commences commercial production of corn-based ethanol at its biorefinery in Nevada, Iowa, USA. |
20/8/2024 | Weltec refurbishes biogas WtE plant near Melbourne, Australia. |
17/9/2024 | Woodland Biofuels plans to build a large, $1.35 billion biofuel plant in St John the Baptist Parish, USA. |
9/2024 | Unconventional Gas Solutions announces commissioning of two of Novilla’s biogas upgrading plants in the US states of Michigan and Wisconsin. The innovative system uses a multi-stage, membrane-based treatment process that tolerates very high H2S concentrations of up to 30,000 ppm. |
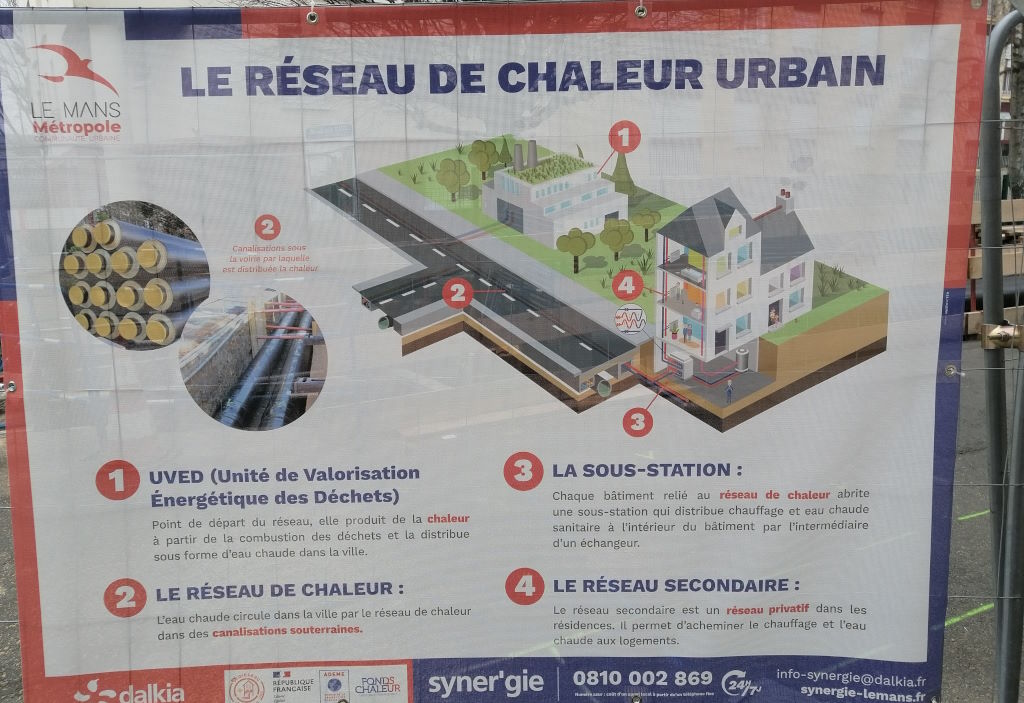
Pros and cons

The main argument in favour of bioenergy is that it decarbonizes the production of energy by relying on feedstock that is self-renewing: the CO2 absorbed by plants as they grow offsets some of the CO2 emitted during the manufacture process. (In addition, Bioenergy Carbon Capture and Storage – BECCS – can be applied.) The case for biofuels is especially powerful for heavy-duty transport systems where the prospects of electrification are limited: jet aircraft, large ships, HGVs, heavy industry (Outokumpu, for example, is building a pelletizing plant that will allow it to replace fossil coke with biomass in its stainless-steel production).
A third advantage of bioenergy is the opportunity to cut waste and advance the circular economy. Waste-to-energy (WtE) in combination with district heating is an example of how to kill two birds with one stone: disposing of waste that would otherwise have to be incinerated or sent to landfills (a major source of methane emissions) while heating homes at lower cost and with fewer emissions. Other benefits include enhanced energy security and a boost to local economies.
Apart from anaerobic digestion, other processes used in WtE include incineration/combustion, which produces heat for district heating or electricity; gasification, which involves heating in a controlled, oxygen-limited environment to produce syngas; and pyrolysis, where biomass is heated to high temperatures in an oxygen-free environment to produce gas for energy or solid and liquid materials that can be refined as bio-oil and chars.
Critics of bioenergy point to the high costs and dependence on subsidies (does bioenergy make sense when the costs of wind and solar have been plummeting?), competition with recycling (diversion of waste that could otherwise be recycled), technological challenges (many processes have been implemented only recently) and the “food versus fuel” argument, which maintains that biofuels take up land that could be used to grow food (an objection that applies more to first-generation than later biofuels).
Stainless steel
Many of the processes used in biofuels are chemical processes, with conditions that make tough demands on materials, both in terms of corrosion and high temperatures. As in many industries, in the early days standard austenitic grades (304 and 316) were applied, but as time went on, recourse was increasingly made to duplex grades. Over time, the trend has been to replace both mild austenitics and higher grades with various duplex grades. Specifically, types 304 and 316 have been replaced by stronger lean duplex grades (LDX 2101 was applied in many ethanol plants); type 317 and some 6% Mo alloys by duplex 2205, and for some applications where 6% Mo (such as 904L) and nickel alloys were applied, superduplex 2507 is now preferred for its better mechanical properties, especially in biodiesel reactor tanks. Duplex grades are now more or less obligatory in process tanks, reactor vessels and digesters. The pretreatment phases of ethanol and biodiesel plants require strong duplex grades (2500 and 2507). Weltec has recently built several biogas plants in which duplex is applied in tanks and biogas reactors. In the tanks, lean duplex type 1.4062/1.4162 is used in the liquid (lower) zone and superduplex 1.4462 (2507) in the gas (upper) zone. The panels are screwed together and pushed up using jacks. For the corrosive, high-pressure environment of convection syngas coolers, Alleima developed a tube where the corrosive syngas is inside the tube and the water/steam is on the outside, in Sanicro® 30 (UNS N08800, EN 1.4558) and Sanicro® 28 (UNS N08028, EN 1.4563).
About this Featured Story
Appearing in the October 2024 issue of Stainless Steel World Magazine, this Featured Story is just one of many insightful articles we publish. Subscribe today to receive 10 issues a year, available monthly in print and digital formats. – SUBSCRIPTIONS TO OUR DIGITAL VERSION ARE NOW FREE.
Every week we share a new Featured Story with our Stainless Steel community. Join us and let’s share your Featured Story on Stainless Steel World online and in print.