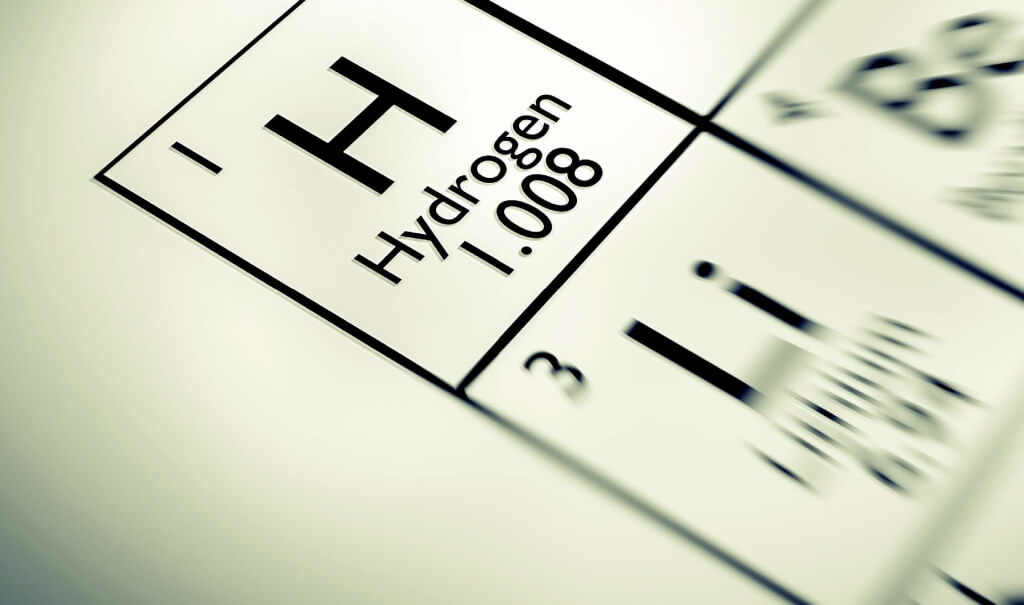
In the quest for a decarbonised society, there is no doubt that as an environmentally friendly fuel source, hydrogen is gaining popularity to become the fuel of the future. The hydrogen revolution is happening well underway.
By Clara Moyano, Parker Hannifin Manufacturing
Hydrogen-based technologies are evolving rapidly, entering the mass market and becoming part of our daily lives. From clean power generation to environmentally friendly cars, the possibilities are endless.
The transportation sector is a prime example of how hydrogen technologies are taking off and making truly sustainable mobility more tangible than ever. Heavy trucks with hydrogenpowered cells are already hitting the roads. Although developing a global hydrogen refuelling infrastructure might take several years or even decades, the commitment of governments around the world to hydrogen economies is accelerating.
The hydrogen challenge
Hydrogen is the most abundant element in nature, and its versatility can offer compelling advantages as an accessible, sustainable, and efficient alternative energy source. However, it can be very damaging for most metallic materials, causing what is known as hydrogen damage or hydrogen attack. Hydrogen degradation is directly connected to its ability to be easily absorbed by metals, coupled with its high mobility on the microstructural level.
Nearly every metallic material can be susceptible to hydrogen damage, and there are several forms of hydrogen degradation. Hydrogen embrittlement cracking is the most common and affects the three main areas of hydrogen industries: production, transportation, and storage.
The hydrogen atoms find preferential places in the structure of the material, modifying its physical properties and mechanical behaviour. The diffusion of hydrogen into the material results in a loss of ductility, making it more brittle and more susceptible to cracking.

Unseen damage

Hydrogen can be a silent assassin, weakening the material slowly and without any clear signs of damage, often leading to critical failure. Three main challenges which determine the effect of hydrogen embrittlement are:
- The environment
- The mechanics of the material
- The microstructure characteristics of the material.
Hydrogen and stress need to be present on a susceptible material for a hydrogen-assisted fracture to occur. Firstly, hydrogen absorption can happen during the production and service stages. Processes such as uncontrolled melting, electroplating, or welding can promote the pre-charge of hydrogen into a given metal. In terms of microstructure, and as a rule of thumb, materials that bestow high mechanical strength or show a great number of defects and inclusions are likely to be more susceptible to this type of failure. The severity of hydrogen embrittlement is also a function of the operating temperature. Low temperatures are the worst-case scenario in terms of material ductility.

The factors that can affect the quality of the microstructure are numerous and widely documented by the materials society. Due to the complexity of the subject, the effect of microstructure (as a major contributing factor to hydrogen behaviour) cannot be evaluated in simplistic terms. Taking one variable in isolation is not enough to guarantee the quality or performance of a given component and can be misleading. For example, a material grade with a ‘perfect chemistry’ or with high levels of a particular ingredient can still result in a very low-quality product.
The common consequences of improper and non-controlled material processing, heat treatment and/or manufacturing operations are high densities of undesirable phases and inclusions in the raw material. These will inevitably lead to premature hydrogen-assisted cracking during service, particularly in demanding H2 environments. Material processing is, therefore, key.
Applications stresses
The mechanics of the application play a major role. Stress states in components can be caused by the presence of residual stresses associated with certain fabrication techniques, or by stresses applied during service. Improper product design and improper installation can cause overloading of stress onto the material.
All of these factors can cause premature failure of components in hydrogen service. When it comes to handling hydrogen, therefore, material and equipment selection becomes, more than ever, an essential ingredient for success.
About the author
As a manufacturer of motion and control technologies, Parker offers products orientated to the hydrogen transportation market, from fuel cell platforms for trucks and buses to hydrogen storage.
Clara Moyano is a Material Scientist for the Instrumentation Products Division Europe and has published several works, including Beyond Stainless Steel: Corrosion Resistant Alloys in the Oil and Gas Industry.